Identifying and quantifying proteolytic events and the natural N terminome by terminal amine isotopic labeling of substrates
Analysis of the sequence and nature of protein N termini has many applications. Defining the termini of proteins for proteome annotation in the Human Proteome Project is of increasing importance. Terminomics analysis of protease cleavage sites in degradomics for substrate discovery is a key new application. Here we describe the step-by-step procedures for performing terminal amine isotopic labeling of substrates (TAILS), a 2- to 3-d (depending on method of labeling) high-throughput method to identify and distinguish protease-generated neo–N termini from mature protein N termini with all natural modifications with high confidence. TAILS uses negative selection to enrich for all N-terminal peptides and uses primary amine labeling-based quantification as the discriminating factor. Labeling is versatile and suited to many applications, including biochemical and cell culture analyses in vitro; in vivo analyses using tissue samples from animal and human sources can also be readily performed. At the protein level, N-terminal and lysine amines are blocked by dimethylation (formaldehyde/sodium cyanoborohydride) and isotopically labeled by incorporating heavy and light dimethylation reagents or stable isotope labeling with amino acids in cell culture labels. Alternatively, easy multiplex sample analysis can be achieved using amine blocking and labeling with isobaric tags for relative and absolute quantification, also known as iTRAQ. After tryptic digestion, N-terminal peptide separation is achieved using a high-molecular-weight dendritic polyglycerol aldehyde polymer that binds internal tryptic and C-terminal peptides that now have N-terminal alpha amines. The unbound naturally blocked (acetylation, cyclization, methylation and so on) or labeled mature N-terminal and neo-N-terminal peptides are recovered by ultrafiltration and analyzed by tandem mass spectrometry (MS/MS). Hierarchical substrate winnowing discriminates substrates from the background proteolysis products and non-cleaved proteins by peptide isotope quantification and bioinformatics search criteria.
This is a preview of subscription content, access via your institution
Access options
Subscribe to this journal
Receive 12 print issues and online access
265,23 € per year
only 22,10 € per issue
Buy this article
- Purchase on SpringerLink
- Instant access to full article PDF
Prices may be subject to local taxes which are calculated during checkout
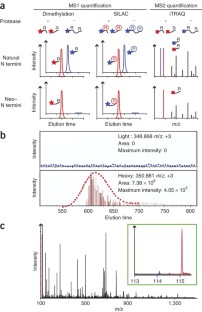
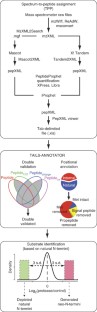
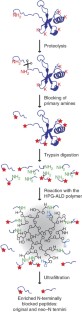

Similar content being viewed by others
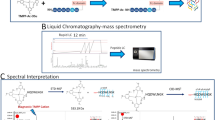
Diagnostic utility of N-terminal TMPP labels for unambiguous identification of clipped sites in therapeutic proteins
Article Open access 30 October 2023
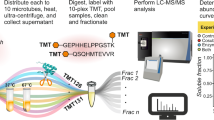
System-wide identification and prioritization of enzyme substrates by thermal analysis
Article Open access 26 February 2021
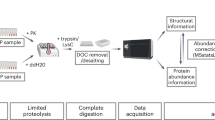
Proteome-wide structural changes measured with limited proteolysis-mass spectrometry: an advanced protocol for high-throughput applications
Article 16 December 2022
References
- Kleifeld, O. et al. Isotopic labeling of terminal amines in complex samples identifies protein N termini and protease cleavage products. Nat. Biotechnol.28, 281–288 (2010). ArticleCASGoogle Scholar
- Kleifeld, O., Doucet, A., Kizhakkedathu, J.N. & Overall, C.M. System-wide proteomic identification of protease cleavage products by terminal amine isotopic labeling of substrates. Protoc. Exchange published online, doi:10.1038/nprot.2010.30 (2010).
- Prudova, A., auf dem Keller, U., Butler, G.S. & Overall, C.M. Multiplex N terminome analysis of MMP-2 and MMP-9 substrate degradomes by iTRAQ-TAILS quantitative proteomics. Mol. Cell. Proteomics9, 894–911 (2010). ArticleCASGoogle Scholar
- auf dem Keller, U., Prudova, A., Gioia, M., Butler, G.S. & Overall, C.M. A statistics-based platform for quantitative N terminome analysis and identification of protease cleavage products. Mol. Cell. Proteomics9, 912–927 (2010). ArticleCASGoogle Scholar
- Hegde, R.S. & Bernstein, H.D. The surprising complexity of signal sequences. Trends Biochem. Sci.31, 563–571 (2006). ArticleCASGoogle Scholar
- McQuibban, G.A. et al. Inflammation dampened by gelatinase A cleavage of monocyte chemoattractant protein-3. Science289, 1202–1206 (2000). ArticleCASGoogle Scholar
- Overall, C.M. & Lopez-Otin, C. Strategies for MMP inhibition in cancer: innovations for the post-trial era. Nat. Rev. Cancer2, 657–672 (2002). ArticleCASGoogle Scholar
- Vergote, D. et al. Proteolytic processing of SDF-1alpha reveals a change in receptor specificity mediating HIV-associated neurodegeneration. Proc. Natl. Acad. Sci. USA103, 19182–19187 (2006). ArticleCASGoogle Scholar
- Overall, C.M. Molecular determinants of metalloproteinase substrate specificity: matrix metalloproteinase substrate binding domains, modules, and exosites. Mol. Biotechnol.22, 51–86 (2002). ArticleCASGoogle Scholar
- Meinnel, T., Serero, A. & Giglione, C. Impact of the N-terminal amino acid on targeted protein degradation. Biol. Chem.387, 839–851 (2006). ArticleCASGoogle Scholar
- Gevaert, K. et al. Exploring proteomes and analyzing protein processing by mass spectrometric identification of sorted N-terminal peptides. Nat. Biotechnol.21, 566–569 (2003). ArticleCASGoogle Scholar
- McDonald, L., Robertson, D.H., Hurst, J.L. & Beynon, R.J. Positional proteomics: selective recovery and analysis of N-terminal proteolytic peptides. Nat. Methods2, 955–957 (2005). ArticleCASGoogle Scholar
- Kuhn, K. et al. Isolation of N-terminal protein sequence tags from cyanogen bromide cleaved proteins as a novel approach to investigate hydrophobic proteins. J. Proteome Res.2, 598–609 (2003). ArticleCASGoogle Scholar
- McDonald, L. & Beynon, R.J. Positional proteomics: preparation of amino-terminal peptides as a strategy for proteome simplification and characterization. Nat. Protoc.1, 1790–1798 (2006). ArticleCASGoogle Scholar
- Mahrus, S. et al. Global sequencing of proteolytic cleavage sites in apoptosis by specific labeling of protein N termini. Cell134, 866–876 (2008). ArticleCASGoogle Scholar
- Van Damme, P., Arnesen, T. & Gevaert, K. Protein alpha-n-acetylation studied by N-terminomics. FEBS J. published online, doi:10.1111/j.1742-4658.2011.08230.x. (7 July 2011).
- Overall, C.M. & Blobel, C.P. In search of partners: linking extracellular proteases to substrates. Nat. Rev. Mol. Cell Biol.8, 245–257 (2007). ArticleCASGoogle Scholar
- Doucet, A., Butler, G.S., Rodriguez, D., Prudova, A. & Overall, C.M. Metadegradomics: toward in vivo quantitative degradomics of proteolytic post-translational modifications of the cancer proteome. Mol. Cell Proteomics7, 1925–1951 (2008). ArticleCASGoogle Scholar
- Polevoda, B. & Sherman, F. Nalpha -terminal acetylation of eukaryotic proteins. J. Biol. Chem.275, 36479–36482 (2000). ArticleCASGoogle Scholar
- Puente, X.S., Sanchez, L.M., Overall, C.M. & Lopez-Otin, C. Human and mouse proteases: a comparative genomic approach. Nat. Rev. Genet.4, 544–558 (2003). ArticleCASGoogle Scholar
- Turk, B. Targeting proteases: successes, failures and future prospects. Nat. Rev. Drug Discov.5, 785–799 (2006). ArticleCASGoogle Scholar
- Lopez-Otin, C. & Overall, C.M. Protease degradomics: a new challenge for proteomics. Nat. Rev. Mol. Cell Biol.3, 509–519 (2002). ArticleCASGoogle Scholar
- Ji, C., Guo, N. & Li, L. Differential dimethyl labeling of N termini of peptides after guanidination for proteome analysis. J. Proteome Res.4, 2099–2108 (2005). ArticleCASGoogle Scholar
- Dormeyer, W., Mohammed, S., Breukelen, B., Krijgsveld, J. & Heck, A.J. Targeted analysis of protein termini. J. Proteome Res.6, 4634–4645 (2007). ArticleCASGoogle Scholar
- Schilling, O. & Overall, C.M. Proteomic discovery of protease substrates. Curr. Opin. Chem. Biol.11, 36–45 (2007). ArticleCASGoogle Scholar
- Timmer, J.C. et al. Profiling constitutive proteolytic events in vivo. Biochem. J.407, 41–48 (2007). ArticleCASGoogle Scholar
- Enoksson, M. et al. Identification of proteolytic cleavage sites by quantitative proteomics. J. Proteome Res.6, 2850–2858 (2007). ArticleCASGoogle Scholar
- Guo, L. et al. A proteomic approach for the identification of cell-surface proteins shed by metalloproteases. Mol. Cell Proteomics1, 30–36 (2002). ArticleCASGoogle Scholar
- Dix, M.M., Simon, G.M. & Cravatt, B.F. Global mapping of the topography and magnitude of proteolytic events in apoptosis. Cell134, 679–691 (2008). ArticleCASGoogle Scholar
- Staes, A. et al. Improved recovery of proteome-informative, protein N-terminal peptides by combined fractional diagonal chromatography (COFRADIC). Proteomics8, 1362–1370 (2008). ArticleCASGoogle Scholar
- Van Damme, P. et al. Caspase-specific and nonspecific in vivo protein processing during Fas-induced apoptosis. Nat. Methods2, 771–777 (2005). ArticleCASGoogle Scholar
- Van Damme, P. et al. Analysis of protein processing by N-terminal proteomics reveals novel species-specific substrate determinants of granzyme B orthologs. Mol. Cell. Proteomics8, 258–272 (2008). ArticleGoogle Scholar
- Vande Walle, L. et al. Proteome-wide identification of HtrA2/Omi substrates. J. Proteome Res.6, 1006–1015 (2007). ArticleCASGoogle Scholar
- Wold, F. In vivo chemical modification of proteins (post-translational modification). Annu. Rev. Biochem.50, 783–814 (1981). ArticleCASGoogle Scholar
- Dean, R.A. & Overall, C.M. Proteomics discovery of metalloproteinase substrates in the cellular context by iTRAQ labeling reveals a diverse MMP-2 substrate degradome. Mol. Cell. Proteomics6, 611–623 (2007). ArticleCASGoogle Scholar
- Dean, R.A. et al. Identification of candidate angiogenic inhibitors processed by matrix metalloproteinase 2 (MMP-2) in cell based proteomic screens: disruption of vascular endothelial growth factor (VEGF)/heparin Affin regulatory peptide (Pleiotrophin) and VEGF/connective tissue growth factor angiogenic inhibitory complexes by MMP-2 proteolysis. Mol. Cell Biol.27, 8454–8465 (2007). ArticleCASGoogle Scholar
- Butler, G.S., Dean, R.A., Tam, E.M. & Overall, C.M. Pharmacoproteomics of a metalloproteinase hydroxamate inhibitor in breast cancer cells: dynamics of matrix metalloproteinase-14 (MT1-MMP) mediated membrane protein shedding. Mol. Cell Biol.28, 4896–4914 (2008). ArticleCASGoogle Scholar
- Hsu, J.L., Huang, S.Y., Chow, N.H. & Chen, S.H. Stable-isotope dimethyl labeling for quantitative proteomics. Anal. Chem.75, 6843–6852 (2003). ArticleCASGoogle Scholar
- Metz, B. et al. Identification of formaldehyde-induced modifications in proteins: reactions with model peptides. J. Biol. Chem.279, 6235–6243 (2004). ArticleCASGoogle Scholar
- Boersema, P.J., Raijmakers, R., Lemeer, S., Mohammed, S. & Heck, A.J. Multiplex peptide stable isotope dimethyl labeling for quantitative proteomics. Nat. Protoc.4, 484–494 (2009). ArticleCASGoogle Scholar
- Pichler, P. et al. Peptide labeling with isobaric tags yields higher identification rates using iTRAQ 4-plex compared to TMT 6-plex and iTRAQ 8-plex on LTQ Orbitrap. Anal. Chem.82, 6549–6558 (2010). ArticleCASGoogle Scholar
- Thompson, A.J. et al. Characterization of protein phosphorylation by mass spectrometry using immobilized metal ion affinity chromatography with on-resin beta-elimination and Michael addition. Anal. Chem.75, 3232–3243 (2003). ArticleCASGoogle Scholar
- Molina, H. et al. Temporal profiling of the adipocyte proteome during differentiation using a five-plex SILAC based strategy. J. Proteome Res.8, 48–58 (2009). ArticleCASGoogle Scholar
- Gioia, M., Foster, L.J. & Overall, C.M. Cell-based identification of natural substrates and cleavage sites for extracellular proteases by SILAC proteomics. Methods Mol. Biol.539, 131–153 (2009). ArticleCASGoogle Scholar
- Heinecke, N.L., Pratt, B.S., Vaisar, T. & Becker, L. PepC: proteomics software for identifying differentially expressed proteins based on spectral counting. Bioinformatics26, 1574–1575 (2010). ArticleCASGoogle Scholar
- Lu, P., Vogel, C., Wang, R., Yao, X. & Marcotte, E.M. Absolute protein expression profiling estimates the relative contributions of transcriptional and translational regulation. Nat. Biotechnol.25, 117–124 (2007). ArticleCASGoogle Scholar
- Schilling, O. & Overall, C.M. Proteome-derived, database-searchable peptide libraries for identifying protease cleavage sites. Nat. Biotechnol.26, 685–694 (2008). ArticleCASGoogle Scholar
- Zhang, R. & Regnier, F.E. Minimizing resolution of isotopically coded peptides in comparative proteomics. J. Proteome Res.1, 139–147 (2002). ArticleCASGoogle Scholar
- Guo, K., Ji, C. & Li, L. Stable-isotope dimethylation labeling combined with LC-ESI MS for quantification of amine-containing metabolites in biological samples. Anal. Chem.79, 8631–8638 (2007). ArticleCASGoogle Scholar
- Higdon, R. & Kolker, E. A predictive model for identifying proteins by a single peptide match. Bioinformatics23, 277–280 (2007). ArticleCASGoogle Scholar
- Keller, A., Eng, J., Zhang, N., Li, X.J. & Aebersold, R. A uniform proteomics MS/MS analysis platform using open XML file formats. Mol. Syst. Biol.1, 2005.0017 (2005). ArticleGoogle Scholar
- Perkins, D.N., Pappin, D.J., Creasy, D.M. & Cottrell, J.S. Probability-based protein identification by searching sequence databases using mass spectrometry data. Electrophoresis20, 3551–3567 (1999). ArticleCASGoogle Scholar
- Craig, R. & Beavis, R.C. TANDEM: matching proteins with tandem mass spectra. Bioinformatics20, 1466–1467 (2004). ArticleCASGoogle Scholar
- Elias, J.E., Haas, W., Faherty, B.K. & Gygi, S.P. Comparative evaluation of mass spectrometry platforms used in large-scale proteomics investigations. Nat. Methods2, 667–675 (2005). ArticleCASGoogle Scholar
- Searle, B.C., Turner, M. & Nesvizhskii, A.I. Improving sensitivity by probabilistically combining results from multiple MS/MS search methodologies. J. Proteome Res.7, 245–253 (2008). ArticleCASGoogle Scholar
- Shteynberg, D. et al. iProphet: Multi-level integrative analysis of shotgun proteomic data improves peptide and protein identification rates and error estimates. Mol. Cell. Proteomics. published online, doi:10.1074/mcp.M111.007690 (2011).
- Elias, J.E. & Gygi, S.P. Target-decoy search strategy for increased confidence in large-scale protein identifications by mass spectrometry. Nat. Methods4, 207–214 (2007). ArticleCASGoogle Scholar
- Choi, H. & Nesvizhskii, A.I. Semisupervised model-based validation of peptide identifications in mass spectrometry-based proteomics. J. Proteome Res.7, 254–265 (2008). ArticleCASGoogle Scholar
- Oliveros, J.C. VENNY. An interactive tool for comparing lists with Venn Diagrams. http://bioinfogp.cnb.csic.es/tools/venny/index.html (2007).
- Butler, G.S. & Overall, C.M. Proteomic identification of multitasking proteins in unexpected locations complicates drug targeting. Nat. Rev. Drug Discov.8, 935–948 (2009). ArticleCASGoogle Scholar
- Butler, G.S. & Overall, C.M. Updated biological roles for matrix metalloproteinases and new 'intracellular' substrates revealed by degradomics. Biochemistry48, 10830–10845 (2009). ArticleCASGoogle Scholar
- Lange, P.F. & Overall, C.M. TopFIND, a knowledgebase linking protein termini with function. Nat. Meth.8, 703–704 (2011). ArticleCASGoogle Scholar
- Chevallet, M., Luche, S. & Rabilloud, T. Silver staining of proteins in polyacrylamide gels. Nat. Protoc.1, 1852–1858 (2006). ArticleCASGoogle Scholar
- Rappsilber, J., Ishihama, Y. & Mann, M. Stop and go extraction tips for matrix-assisted laser desorption/ionization, nanoelectrospray, and LC/MS sample pretreatment in proteomics. Anal. Chem.75, 663–670 (2003). ArticleCASGoogle Scholar
- Rappsilber, J., Mann, M. & Ishihama, Y. Protocol for micro-purification, enrichment, pre-fractionation and storage of peptides for proteomics using StageTips. Nat. Protoc.2, 1896–1906 (2007). ArticleCASGoogle Scholar
- Boja, E.S. & Fales, H.M. Overalkylation of a protein digest with iodoacetamide. Anal. Chem.73, 3576–3582 (2001). ArticleCASGoogle Scholar
- Nielsen, M.L. et al. Iodoacetamide-induced artifact mimics ubiquitination in mass spectrometry. Nat. Methods5, 459–460 (2008). ArticleCASGoogle Scholar
- Gidley, M.J. & Sanders, J.K. Reductive methylation of proteins with sodium cyanoborohydride. Identification, suppression and possible uses of N-cyanomethyl by-products. Biochem. J.203, 331–334 (1982). ArticleCASGoogle Scholar
- Jentoft, N. & Dearborn, D.G. Labeling of proteins by reductive methylation using sodium cyanoborohydride. J. Biol. Chem.254, 4359–4365 (1979). CASPubMedGoogle Scholar
- Hwang, S.I. et al. Direct cancer tissue proteomics: a method to identify candidate cancer biomarkers from formalin-fixed paraffin-embedded archival tissues. Oncogene26, 65–76 (2007). ArticleCASGoogle Scholar
- Fu, Q. & Li, L. De novo sequencing of neuropeptides using reductive isotopic methylation and investigation of ESI QTOF MS/MS fragmentation pattern of neuropeptides with N-terminal dimethylation. Anal. Chem.77, 7783–7795 (2005). ArticleCASGoogle Scholar
- Ding, Y., Choi, H. & Nesvizhskii, A.I. Adaptive discriminant function analysis and reranking of MS/MS database search results for improved peptide identification in shotgun proteomics. J. Proteome Res.7, 4878–4889 (2008). ArticleCASGoogle Scholar
- Wessa, P. Free Statistics Software, Office for Research Development and Education, version 1.1.23-r6, http://www.wessa.net/ (2010).
- Shevchenko, A., Tomas, H., Havlis, J., Olsen, J.V. & Mann, M. In-gel digestion for mass spectrometric characterization of proteins and proteomes. Nat. Protoc.1, 2856–2860 (2006). ArticleCASGoogle Scholar
- Ong, S.E. & Mann, M. A practical recipe for stable isotope labeling by amino acids in cell culture (SILAC). Nat. Protoc.1, 2650–2660 (2006). ArticleCASGoogle Scholar
- Ishihama, Y., Rappsilber, J. & Mann, M. Modular stop and go extraction tips with stacked disks for parallel and multidimensional Peptide fractionation in proteomics. J. Proteome Res.5, 988–994 (2006). ArticleCASGoogle Scholar
Author information
- Oded Kleifeld, Ulrich auf dem Keller & Magda Gioia Present address: Present addresses: Department of Biochemistry and Molecular Biology, Monash University, Clayton, Melbourne, Australia (O.K.); Ottawa Institute of Systems Biology, Department of Biochemistry, Microbiology and Immunology, University of Ottawa, Ottawa, Ontario, Canada (A.D); Institute of Cell Biology ETH Zurich, Zurich, Switzerland (U.a.d.K.); Department of Experimental Medicine and Biochemical Sciences, Università di Roma Tor Vergata, Roma, Italy (M.G.).,
- Oded Kleifeld, Alain Doucet, Anna Prudova, Ulrich auf dem Keller and Magda Gioia: These authors contributed equally to this work.
- chris.overall@ubc.ca
Authors and Affiliations
- Department of Biochemistry and Molecular Biology, University of British Columbia, Vancouver, British Columbia, Canada Oded Kleifeld, Alain Doucet, Anna Prudova, Ulrich auf dem Keller, Magda Gioia & Christopher M Overall
- Department of Oral Biological and Medical Sciences, University of British Columbia, Vancouver, British Columbia, Canada Oded Kleifeld, Alain Doucet, Anna Prudova, Ulrich auf dem Keller, Magda Gioia & Christopher M Overall
- Department of Pathology and Laboratory Medicine, University of British Columbia, Vancouver, British Columbia, Canada Jayachandran N Kizhakkedathu
- Department of Chemistry, University of British Columbia, Vancouver, British Columbia, Canada Jayachandran N Kizhakkedathu
- Oded Kleifeld